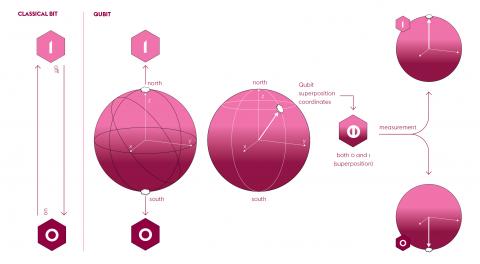
Quantum ambitions: positioning France at the cutting edge of technology
(This article was originally published in L'Edition No.18)
An overview of some of the projects that are part of France’s national quantum strategy, in which Université Paris-Saclay researchers are involved.
The President of Université Paris-Saclay, Sylvie Retailleau announced in the lead-up to the conference “Ambitions quantiques: renforcer le leadership de Europe” (Quantum ambitions: strengthening Europe’s leadership), which took place on 21st February 2022 as part of France’s EU presidency: “Quantum technology development is now at a crossroads of opportunities and challenges”.
With their strong potential for disruption and economic and societal transformation, quantum technologies are one of the most competitive research areas, located at the interface between basic and applied research. Their potential impact, whether in new approaches to high-energy physics, quantum chemistry, quantum computing, quantum cryptography or quantum sensors, explains why these technologies are the subject of an intense global race between research laboratories, emerging start-ups and digital giants.
A dedicated national strategy
As part of the Quantum Plan – the French quantum strategy – launched by the French President Emmanuel Macron on 21st February 2021 at the Saclay plateau, the Quantum Technologies Priority Research Programmes and Equipment (PEPR) is one of the first systems to be created to support world-class research in this field. Directed by the CEA (the French Alternative Energies and Atomic Energy Commission), the CNRS (French National Centre for Scientific Research) and Inria (the French National Institute for Research in Digital Science and Technology), its objective is to place France at the forefront of this development and consolidate French leadership.
With a budget of 150 million euros, the PEPR Quantum Technologies programme supports projects that focus on six areas: quantum accelerators and simulators; scaling up the quantum computer; quantum sensors; post-quantum cryptography; quantum communications, and enabling technologies. The programme also covers four multi-disciplinary focuses, which are: developing human capital, strengthening technological infrastructures, improving the entrepreneurial environment and strengthening inter-disciplinary collaboration and risk-taking in upstream research. In early March 2022, the first ten projects involving laboratories and start-ups from the Paris-Saclay, Grenoble and Paris-Centre quantum clusters, and two EquipEx+ structural facilities were selected for funding. Researchers from Université Paris-Saclay are involved in seven of these projects as well as in EquipEx+ e-DIAMANT, directed by the École Normale Supérieure Paris-Saclay, which will create the entire diamond manufacturing chain for quantum applications.
Qubits and quantum information
A research focus, the qubit is the quantum counterpart of the traditional computer bit. While the traditional bit transcribes information in a binary way (0 or 1), according to whether or not there is an electrical current present and electric charges are circulating in the system, the qubit is generated by quantum objects (atoms, particles, spins, etc.) integrated in the system and its state (or value) is not as clear-cut. This state is defined as a superposition of the 0 and 1 states and is expressed by a mathematical equation that shows an infinite number of possible values.
As a result of this, the information contained in the qubits is extremely rich. It is this richness that has created much enthusiasm about the quantum computer, which is expected to yield a tenfold increase in computing capacity. In such a system, computational operations are performed by acting on the qubits by means of physical devices. However, this is where the problem lies. Quantum superposition, and quantum effects more generally, are fragile and very sensitive to the environment and to outside disturbances that inevitably effect physical systems. Quantum superposition can quickly be collapsed and lead to qubit decoherence. This decoherence makes the qubits illegible and this affects the quantum information: it is deteriorated by an accumulation of random errors.
The importance of coherence
One of the challenges is to succeed in prolonging the coherence of qubits as much as possible, i.e.: their stability and the duration during which it is possible to carry out calculations. To do this, several types of qubits are being researched. Their characteristics vary according to the type of quantum object on which they are based: superconductors, trapped atoms, photons, nuclear spins, electron spins, etc. However, despite numerous announcements concerning the ever-increasing number of qubits reached by the quantum processors developed by the industrial giants, there is still no hardware architecture capable of generating qubits protected against decoherence.
The most common approach to combat this is the use of redundancy: instead of being encoded in a single qubit, the quantum information is written in a large number of qubits. These ‘physical’ qubits, which form a ‘logical’ or ‘protected’ qubit, preserve the quantum information. Although promising, this approach requires a very large number of physical qubits to form a single logical qubit. However, the most optimistic predictions agree that 1,000 physical qubits are needed to obtain 1 logical qubit in order to succeed in running algorithms with low demand, and with error rates of less than 1% on all operations.
More robust qubits for quantum computing
The RobustSuperQ project aims to accelerate French R&D on hybrid superconducting qubits protected by construction against decoherence. In the next five years, the plan is to develop new generation superconducting qubits as well as a new type of quantum processor, which is corrected, high-fidelity, controllable and measurable, and composed of very robust physical qubits, a small number of which form a logical qubit.
In collaboration with all the academic and private participants of the quantum circuit community (including the manufacturer ATOS and the start-ups C12 and Alice & Bob), the Quantronics and Nanoelectronics Groups in the Condensed Matter Physics Laboratory (SPEC – Univ. Paris-Saclay, CEA, CNRS), teams from the Institute of Theoretical Physics (IPhT – Univ. Paris-Saclay, CNRS, CEA) and the Theory team of the Solid State Physics Laboratory (LPS – Univ. Paris-Saclay, CNRS) will research three development areas in parallel: dynamically stabilised “cat” qubits, a hybrid architecture based on highly coherent electronuclear spins magnetically coupled to superconducting circuits, and superconducting qubits that are topologically protected against decoherence.
Towards new quantum simulators
Although a universal quantum computer is still far off, there are platforms that are already capable of carrying out complex quantum simulations that are inaccessible using traditional computing methods. Some are based on arrays of atoms trapped by a system of “optical tweezers”, brought into interaction using a laser that plunges them into a very high level of excitation called the “Rydberg state”. Others use ultra-cold atoms, cooled to temperatures approaching absolute zero (0 K or -273.15 °C) – interacting in optical gratings using laser beams.
The objective of the QubitAF project is to improve these two platform types by increasing the number of atoms manipulated, and to characterise and certify the results. The three experimental groups involved, including Charles Fabry Laboratory (LCF – Univ. Paris-Saclay, IOGS, CNRS), and the start-up Pasqal from the LCF, will have the goal of developing a new generation of Rydberg simulator, as well as new types of cold atom simulators. The aim is also to develop new tools to diagnose the quantum properties of these systems, such as quantum entanglement or quantum correlation.
Improving fault tolerance
While a large number of qubits and sufficient coherence are important elements, it is also necessary for the system to be able to maintain a low error rate. This requires the implementation of error correction processes: correcting codes. Their role is to relocate the quantum information from one part of the system to other physical systems, to better protect it from background noise.
The NISQ2LSQ project, involving around 20 academic teams including SPEC’s Quantronics and teams at LCF and the Centre for Nanoscience and Nanotechnology (C2N – Univ. Paris-Saclay, CNRS, Univ. de Paris), as well as the start-ups Alice&Bob and Quandela from C2N, aims to develop new error correction strategies, thereby obtaining fault-tolerant quantum computers. Research will focus on bosonic codes, photonic codes and LDPC (Low-Density Parity-Check) codes, which will be explored on two types of physical platforms: superconducting circuits and photonic circuits. Within five years, the partners aim to build a prototype superconducting quantum processor based on cat qubits, which is more fault-tolerant, to define measurement-based computing architectures exploiting photonic codes, and to determine the elements necessary for their construction.
More efficient and advanced quantum algorithms
To obtain a real quantum advantage, it is not enough to build a machine that works according to quantum principles, you also need to develop efficient algorithms that exploit the specificities of quantum mechanics and are capable of resolving problems efficiently. One of the challenges is to introduce new algorithmic techniques with real proven advantages, but also to enrich the potential application areas of quantum information processing.
More than 20 academic and private partners will work on the EPiQ project to develop quantum algorithms in the areas of optimisation, machine learning, simulation of physical systems or chemistry, both for NISQ (Noisy Intermediate-Scale Quantum) machines – machines with 50 to 100 qubits without reliable error correction but still capable of performing tasks superior to conventional computers – and for fault-tolerant machines. The QUACS project team from the Formal Methods Laboratory (LMF – Univ. Paris-Saclay, CNRS, ENS Paris-Saclay, CentraleSupélec, Inria), teams from the Systems and Technology Integration Laboratory (List – Univ. Paris-Saclay, CEA), the Interdisciplinary Laboratory of Digital Sciences (LISN – Univ. Paris-Saclay, CEA, CNRS) and IPhT will focus on developing new quantum algorithms, better defining the architectures and languages of current machines, and simulating them to better understand them.
Quantum cryptography and communication: better resistance to attacks
With progress made in quantum computing, the whole field of cryptography and communication security is being challenged, particularly the web and its TLS protocol (the browser padlock). While this security is based on the robustness of cryptographic algorithms that are difficult to solve using a conventional computer, they would offer little protection from a quantum computer. Faced with such threats, partners working on the project PQ-TLS, including the Mathematics Laboratory of Versailles (LMV – Univ. Paris-Saclay, UVSQ, CNRS) and the LMF, aim to develop new encryption and signature schemas to bring cryptography into the post-quantum era and make protocols resistant to attack.
Quantum key distribution is also based essentially on the assumption that the devices used perform exactly the operations envisaged by the protocols. In practice, this is difficult to verify. The objective of the DIQKD project, which includes a team from the IPhT, is to produce the first “black box” quantum key distribution prototype, capable of guaranteeing unprecedented confidentiality of communications over a greater distance, and of resisting quantum attacks for devices that are not or only partially characterised.
Another project, QcommTestbed, in which C2N is involved, aims to provide academic and industrial teams with a flexible and scalable national test infrastructure for quantum communication applications. This testbed will be used to progress from laboratory demonstrations to field prototypes and commercial products.
These are all elements that should serve to shift the development of quantum technologies into a new era.
Anticipating the quantum computer’s response
At IJCLab, Denis Lacroix and his team are designing algorithms capable of describing sets of interacting particles. They are testing their calculations in anticipation of future quantum computers.
A theoretical physicist at the Irène-Joliot Curie Physics of Two Infinities Laboratory (IJCLab – Univ. Paris-Saclay, CNRS, Univ. Paris Cité), Denis Lacroix studies the strong interactions between protons and neutrons in atomic nuclei, which he usually models on conventional computers. “But with a large number of particles interacting, matrices quickly reach critical dimensions and explode,” explains the researcher. Such limitations can potentially be eliminated with a quantum computer.
With his team, Denis Lacroix analyses the way in which it will be possible to use these machines. “We are preparing for the future, for the day when they will be ready.” The researcher is developing methods and algorithms that aim to resolve problems that conventional computers cannot tackle. In his latest work, he is interested in the total spin of an n-body system. “We developed an algorithm that can sort the total spin of a set of particles, i.e. filter this disordered system to retain only those spins that are in a given orientation.” He also sought to calculate the energy of a quantum system in different states. “The method is iterative and hybrid: the parameters of the system are varied on a conventional computer, then its energy is calculated on a quantum computer using a wave function that we have prepared, and the parameters are readjusted as we go along.”
To test the developed algorithms, the researcher uses a quantum computer simulator made available free of charge by IBM via the Cloud. “We are working ‘as if’ we were working with a real quantum computer, without having the most recent developments.” In the future, the researcher plans to push the description of the nuclei to the limit: “It is about going towards larger and larger nuclei, and seeing how the system evolves, how it vibrates, moves, and goes as far as fission.”
Publication :
- Guzman E. A. R., Lacroix D. Accessing ground-state and excited-state energies in a manybody system after symmetry restoration using quantum computers. Phys. Rev. C 105, 024324, (2022).
A highly sensitive microwave photon detector
At the Condensed Matter Physics Laboratory (SPEC – Univ. Paris-Saclay, CEA, CNRS), Emmanuel Flurin and his colleagues are pushing the limits of magnetic resonance with an ingenious microwave photon counter capable of detecting a very small number of electron spins.
The device developed by SPEC scientists combines superconducting quantum circuits and electron spins in solids, and is applicable at cryogenic temperatures. Spin is a system with two distinct quantum levels – basic and excited – which switch from one state to the other via the absorption/emission of a single photon whose energy is in the microwave range. Since de-excitation only occurs spontaneously after a very long time, it is possible to accelerate it by using a superconducting resonant cavity. The emitted photons are then collected by the microwave photon counter, which consists of a superconducting qubit capable of irreversibly absorbing the photon. The qubit’s subsequent measurement in its excited state signals the presence of the photon and results in a detector click. “Counting spins is like reading the superconducting qubit in its excited state,” sums up Emmanuel Flurin. The team recently provided a proof of principle by detecting a small set of electron spins in silicon.
The INGENIOUS project, awarded an ERC Starting Grant in 2021 and led by Emmanuel Flurin, is a continuation of this research. “We wanted to develop new generations of photon counters capable of achieving sufficient sensitivity to detect an individual electron spin.” This would open up applications in quantum computing. The work will move to electron spins of erbium (Er3+) ions in tungsten oxide crystal, for which the team recently observed coherence times of 23 ms. “If we can manipulate an individual electron spin, we can also manipulate nuclear spins, whose coherence times are potentially even longer.”
Publication :
- Albertinale, E. et al. Detecting spins by their fluorescence with a microwave photon counter. Nature 600, 434–438 (2021).