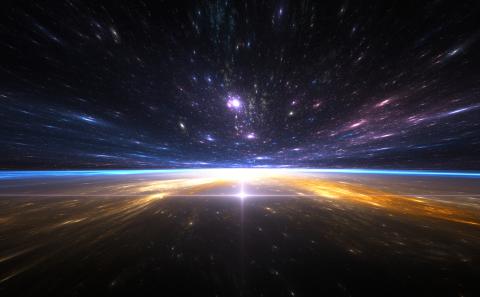
Low-energy cosmic rays: between mysteries and applications
This article was originally published in L'Édition n.22
Cosmic rays, produced by extraordinary events such as supernovae, pass through the Universe, and thus through the Solar System and the Earth. What are these particles, which can have very high or very low energies, and what observations and applications are possible using these cosmic rays?
Cosmic radiation defines the flow of subatomic particles and atomic nuclei circulating in the Universe. The vast majority of these cosmic rays are composed of protons (89%) and helium nuclei (around 9%). The hypothesis of cosmic rays was first put forward by German physicist Albert Gockel in 1909, and confirmed by Victor Hess three years later. While aboard a hot-air balloon, the Austrian physicist discovered that the level of ionisation in the atmosphere at an altitude of 5,000 metres was three times higher than at sea level. His deductions were breath-taking for the time, as they meant that radiation from outer space was colliding with the Earth. These rays travel at close to the speed of light, penetrating the different layers of the Earth's atmosphere, which repel the majority of them. Even today, the scientific community still ponders the origin of the radiation that passes through the Universe. What is even more fascinating is that the detection and observation of these particles have given rise to a host of applications that many researchers at Université Paris-Saclay and elsewhere have seized on.
Cosmic rays or cosmic particles?
"Cosmic radiation is actually a misnomer," explains Vincent Tatischeff, Director of Research at the Laboratory of the Physics of the two Infinities - Irène Joliot-Curie (IJCLab - Univ. Paris-Saclay, Univ. Paris Cité, CNRS). "Cosmic rays or radiation are historical terms, used for a long time (and still used today, incorrectly) before the term cosmic particle flux, which corresponds more to what we can observe. These cosmic particles represent a non-negligible component of the galaxy, in the sense that their energy density - the amount of their energy calculated per unit volume - is quite comparable to the amount of gas thermal energy or to the energy of the galactic magnetic field. This balance is due to the exchange of energy between cosmic particles and other constituents of the interstellar medium."
So what about the composition of these cosmic particles? "We are dealing with a whole spectrum," says the astrophysicist. While protons account for about 89% of the cosmic particle population, many atomic nuclei have also been observed. "Helium atoms, known as alpha particles, account for around 9% of cosmic particles. We also find heavier nuclei, such as carbon, nitrogen and oxygen, which make up approximately 1% of cosmic particles. Lastly, electrons typically make up 1% of this composition," explains Vincent Tatischeff.
"A true wonder of nature"
"We are still trying to understand where these particles come from," says the Director of Research."The current hypothesis of the scientific community is that these particles are accelerated during events such as supernovae. It is possible that they come from other sources, but the shock waves generated by these events contain a particle acceleration mechanism, which was more or less predicted by Enrico Fermi in the 20th century”. Once accelerated, cosmic particles interact with the gases and photons that make up their environment, producing gamma rays that can be observed from Earth using gamma-ray telescopes. The presence of cosmic particles accelerated by exploding stars can be established by the "gamma signatures" they produce. "There are other possible sources of cosmic radiation, such as winds from massive stars, but the current consensus is that most of this radiation comes from supernova star explosions," concludes the astrophysicist.
The energy distribution of the cosmic particles studied by astrophysicists is vast. "The energy spectrum of these particles spans around 14 orders of magnitude from the mega-electronvolt (MeV, 106 eV) up to 1020 eV," says Vincent Tatischeff. "In terms of particle flux (the number of particles observed per square centimetre, per second and per unit of energy), our observations span 30 orders of magnitude. Cosmic particles are a true wonder of nature, their flow as a function of energy form a power law covering 30 orders of magnitude from low energies to extreme energies. This colossal gap is, for example, that which separates the picosecond (10-12 seconds) from the age of the Universe!"
The mystery of low-energy cosmic particles
Ranging from the mega-electronvolt to the giga-electronvolt (GeV, 109 eV), low-energy cosmic rays play a key role in the formation of new stars in the galaxy. "Low-energy cosmic particles are responsible for the ionisation of gas in the galaxy. There are clouds of weakly ionised gas in the galaxy, which collapse in on themselves, creating new stars," explains Vincent Tatischeff. "But the ionization of these clouds by low-energy cosmic rays slows or even prevents the gravitational collapse at the origin of star formation. The presence of this cosmic radiation regulates the collapse of gas clouds, ultimately leading to the creation of new stars."
The existence of these low-energy cosmic particles remained a mystery to the scientific community for a very long time. The reason was solar modulation, a phenomenon that describes the particle winds emitted by the Sun into the Solar System, of which the Earth is a part. These winds fill a cavity formed around the planetary system, repelling low-energy cosmic rays: the heliosphere. "Solar winds exert a pressure that stops the propagation of low-energy cosmic particles within the Solar System. Since these particles have never reached the Earth, they were unknown to us," explains Vincent Tatischeff. It was not until the Voyager 1 probe left the heliosphere that low-energy cosmic rays were finally measured. In August 2012, 35 years after its launch, the probe reached the farthest reaches of the Sun's influence, at a distance of around 18 billion kilometres. Initial measurements indicate radiation energies close to 3 MeV. "We don't really know what is going on below this energy level. But overall, there is no reason to think that there are suddenly no more lower-energy particles," comments Vincent Tatischeff.
While Voyager 1 is still subject to the star's gravitational pull when it leaves the heliosphere, it is no longer subject to the solar wind. "It is an incredible feat," confesses Vincent Tatischeff. "For the first time, we have obtained a direct measurement of low-energy cosmic rays in the local interstellar medium around the Solar System." This discovery now raises new questions for scientists, particularly concerning the ionisation rates for which low-energy cosmic rays are responsible. "Overall, our observations lead us to believe that there are, on average, more low-energy cosmic rays in the galaxy than locally, where we have been able to measure them," explains the astrophysicist. "This means that the density of cosmic radiation varies from region to region, due to the density of events (such as the explosion of stars into supernovae) creating this radiation." The heliosphere, which encloses the Solar System, is itself enclosed in a local bubble of hot gas which some theories believe also protects its internal regions from low energy cosmic rays. "This would explain the low local density of low-energy cosmic rays measured by the Voyager probes," says Vincent Tatischeff.
Cosmic particles and pyramids
David Attié studies muons in the Division of Electronics, Detectors and Computing for Physics (Dedip - Univ. Paris-Saclay, CEA) of the Institute of Research into the Fundamental Laws of the Universe (Irfu). Muons are part of the Standard Model of particle physics and are produced by the encounter between cosmic rays and the Earth's upper atmosphere. The interaction between a cosmic particle and an atmospheric nucleus gives rise to a shower of new particles, notably pi mesons. Positive and negative muons are then produced by the decay of pi mesons, and pass through the different layers of the atmosphere to reach the Earth's surface. These particles are bombarding the Earth at every moment; the flow of muons reaching sea level is around 170 per square metre per second. Although harmless to humans, it is the penetrating nature of this particle that makes it so special. "For example, a 100-metre-thick rock will absorb almost all the muons that pass through it, but not all of them. And it is this tiny percentage that is particularly interesting," explains David Attié.
In 2012, the physicist began designing a cosmic bench to detect the passage of cosmic particles, including muons, and measure their trajectories. The instrument consists of a hodoscope to detect particle position and trajectories, glittering plastic plates to trigger the device when a particle passes through it, and electronic components so that the collected data can be read. "Lastly, a cosmic bench allows us to study and use natural cosmic radiation, with its low but permanent flux, and avoids the sometimes-restrictive use of particle accelerators," explains David Attié.
Following the design of this instrument, David Attié and his colleagues turned to muon tomography, an imaging technique that uses muons and their penetrative properties to study the interior of targeted structures. In 2015, using the cosmic bench and muon telescopes, Irfu physicists recorded the muon fluxes that passed through the famous water tower at CEA Paris-Saclay. The muographic "snapshots" produced by this experiment showed the castle's internal water level at different times of the year. "We carried out two measurement campaigns, the first when the water tower was full and the second when the tank was being cleaned. For the first time using muon tomography, it is perfectly possible to see the design of the castle's structure and even its container on the images we obtained. We found differences between the two images when the water tower is emptied for cleaning and when it is full," says the researcher.
In parallel with these initial studies, the ScanPyramids project, led by Cairo University in Egypt and the French Heritage Innovation Preservation (HIP) Institute, was launched. Its aim was to study the great Egyptian pyramids and their cavities without drilling new holes. This challenge was seemingly destined for the muon tomography of David Attié and his colleagues. In collaboration with Japanese physicists from Nagoya University, researchers from CEA Paris-Saclay went to Egypt and its seventh wonder of the world, the Great Pyramid of Giza, following their first conclusive observations at the water tower. What was supposed to be a test phase for their new instruments turned into a major discovery.
"On the northeast face of the pyramid is a small chamber, a cavity just a few cubic metres in size already accessible and known to specialists. Our idea was therefore to test our technology on this face, pointing our telescopes at this cavity, to demonstrate that we are able to observe this chamber located a few metres of rock from the outside. Not only were we able to observe this small chamber, we also detected the presence of another, previously unknown cavity," says David Attié. Above the Grand Gallery, on the pyramid's northeast face, CEA scientists confirmed in 2017 the presence of a previously unknown "big void", and then using muon tomography directly inside the pyramid two years later. The Japanese researchers confirmed the discovery with measurements from inside the pyramid; muon tomography had indeed discovered a new chamber, located above the Grand Gallery and close to the small chamber.
This momentous discovery is just one example of the possible applications of muon tomography and the observation of cosmic rays passing through the Universe and the Earth at every moment.
References
- Vieu T., et al., Cosmic ray production in superbubbles, Monthly Notices of the Royal Astronomical Society, vol. 512.
- Elkarmoty, M., et al., Discovery and validation of the North Face Corridor in the Great Pyramid of Giza using non-destructive techniques. Papers of the ECNDT 2023. Research and Review Journal of Nondestructive Testing, Vol. 1(1).
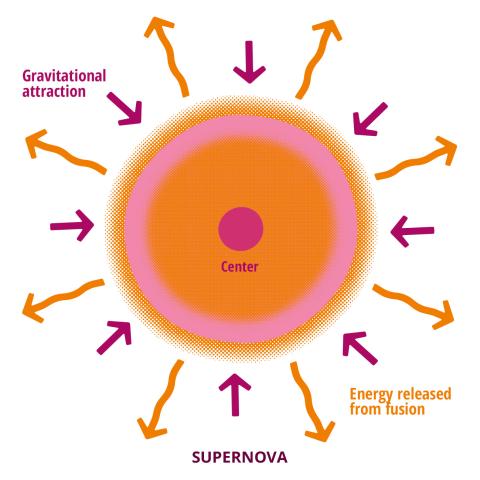
What is a supernova?
A supernova is the spectacular end-of-life process for a star. A star burns up hydrogen – among other – atoms over the course of its existence, which is generally between several million or several billion years. This combustion then triggers nuclear fusion reactions in the star's core, leading to the formation of ever heavier nuclei (from hydrogen to helium, then carbon, oxygen, silicon and iron).
At the centre of the star, where the heaviest elements reside, the pressure is so great that it rivals the forces of gravity and the star begins to collapse. This "iron core" drastically reduces in size in record time, taking with it the star's different internal strata. This collapse causes the star to implode, generating a light of incomparable intensity in the Universe.
The satellite whisperers: portrait of instrument scientists
In the large family of astrophysicists, there is a special profession at the frontier between research and engineering. We take a look at instrument scientists, a position that involves working very closely with satellites.
Arnaud Claret is Director of the Science and Space Instrument Interface Laboratory (LISIS - Univ. Paris-Saclay, CEA) within the Institute of Research into the Fundamental Laws of the Universe (Irfu) of CEA Paris-Saclay. He also occupies a singular role at CEA Paris- Saclay, that of instrument scientist. "I am an astrophysicist but I have an instrument scientist's profile. In other words, roughly speaking, I have one foot in science and the other in technology," says Arnaud Claret. "An instrument scientist's job is to ensure that an instrument's performance is maintained to guarantee the success of a mission. This requires both scientific and technical knowledge. More specifically, I am interested in the effects of space radiation, such as cosmic rays, on onboard satellite instrumentation."
Arnaud Claret is a radiation expert for Irfu's space projects, including the Franco-Chinese low-Earth orbit mission Space-based Multi-band Variable astronomical Objects Monitor (SVOM). "This mission is very interesting for me, not least because it will be located at an exceptionally low orbit of 600 kilometres in altitude. This implies that the satellite will regularly cross the South Atlantic Anomaly." This anomaly is due to the tilt and offset of the Earth's magnetic field in relation to the poles.
The result is that the magnetic field enveloping the Earth is 500 kilometres closer to the ground in the South Atlantic than anywhere else on the planet. This magnetic field acts as a shield, repelling most cosmic rays, particles and solar winds. However, some of these particles remain "trapped" in the magnetic field and spiral in orbit around the planet. "We end up with a magnetic field around the Earth structured like an 'onion' and in concentric shells," describes Arnaud Claret. And trapped particles sometimes remain between these field lines for a very long time. So, a satellite orbiting the Earth at a constant altitude like SVOM will experience this South Atlantic Anomaly at times and, for a certain period, find itself in a "magnetic shell" teeming with particles.
Therein lies the complexity of the SVOM mission for instrument scientists, i.e., how to design instruments whose shielding can cope with this anomaly. And how to manage the "background noise" generated by the regular passage through this area filled with particles of all kinds. "This is all new to me, and pretty exciting. I was able to carry out simulation work using data from different observatories and different satellites that have already made this kind of orbit, to try and predict SVOM's behaviour. In the end, the job is quite similar to that of astrophysicists, who seek to predict their future observations; we have a similar scientific approach. This simulation work gives us the feeling that we are making the instrument our own and developing a certain intimacy with it as we try to predict its operation as accurately as possible," describes Arnaud Claret.