
Understanding cell movement: the secrets of actin networks
Sometimes a whole area of knowledge is concealed in an unexpected area. This is what happened during studies in biophysics carried out as part of the MicMactin project led by Martin Lenz’s team at the Theoretical Physics and Statistical Models Laboratory (LPTMS – Université Paris-Saclay, CNRS). Their results have improved our understanding of the phenomena of cell contraction. A simple initial statistical investigation enabled him to highlight a phenomenon of emergence which could have wide-ranging implications.
As part of the Soft biophysics research group at LPTMS, Martin Lenz and his team work on analysing the basic mechanisms behind the movements of living organisms. Cells in the human body do not remain static. They stretch, contract, deform and some even move within the body under their own power. This movement of cells is essential for the proper functioning of the body. The collective contraction of cells orchestrates muscle movements and, on a smaller scale, the migration of an immune cell is crucial for taking care of an infected site. The mechanisms of these cell movements have been known about and accepted by the scientific community for several decades. However, through his work Martin Lenz has uncovered a detail which could call everything into question.
A mystery hidden in our cells
According to current scientific knowledge, the autonomous movements of a cell are determined by its cytoskeleton, and more precisely by actin. This cytoskeleton is like a microscopic scaffold composed of microscopic beams and cords distributed throughout the cell.
Actin filaments are characterised by not being symmetrical and this property influences the functioning of myosin - the molecular motor at the origin of cellular movement. Depending on how it catches the actin filaments, myosin either pulls them together or apart (see figure). In a striated muscle cell, actin is organised in a bundle and myosin can only bring these filaments together during contraction. However, in other cells, the actin is disorganised. In this case, statistically it is expected that the filaments will contract in 50% of cases and expand in the remaining 50%.
However, in experiments only contraction is observed. Why is the microscopic behaviour of actin not reflected in the macroscopic results obtained? This question led to Martin Lenz laying the foundations of the MicMactin project, for which he was awarded an ERC Starting Grant in 2015.
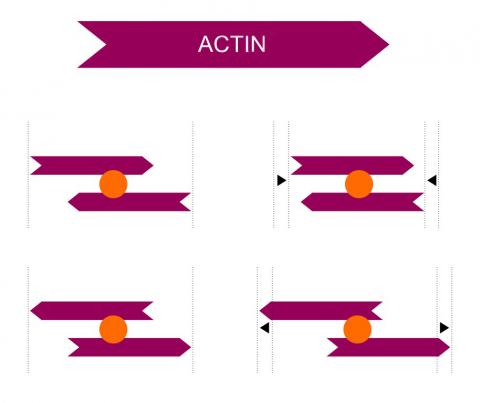

The buckling solution
To try and understand the strange behaviour of the actin, the researcher established the fundamentals of the problem by considering the interactions between several actin filaments. He assumed that actin filaments are like tiny ropes and not like beams. A rope behaves differently depending on whether it is pulled or pushed. It is like a game of tug-of-war. If the players used a beam, it would be possible for each to push it towards the other to see who would be stronger. In contrast, to effectively communicate force with a rope, you have to pull on it. If the rope is pushed, it buckles (see figure) and the effort expended is useless. This difference between extension and contraction leads to a break in symmetry.
Martin Lenz and his team, together with scientists at the University of Chicago, assembled a bundle of actin filaments to myosin - their molecular motor. Under the microscope, they could see small loops forming when actin filaments were pushed towards each other. Their hypothesis was confirmed - actin behaves like a rope. This buckling would explain why microscopic pushing forces are not apparent at the macroscopic level, unlike stretching.
From a bundle to a network
These first results were obtained on well-ordered actin bundles, so the MicMactin team decided to put a molecular motor inside a more disorganised actin network. While the behaviour of actin filaments was predictable near the motor, an intriguing phenomenon occurred on a larger scale in this network. It did not matter whether the engine was contracting or extending locally, as it always resulted in a large-scale contraction (see figure). Contraction was inevitable at the macroscopic level of the cell whatever was happening at the microscopic level. “We were witnessing a phenomenon which physicists love - that of emergence. The behaviour of the overall structure cannot be explained by simply studying the individual properties of its components,” explains Martin Lenz.
Putting it in perspective
In the cells of striated muscles, actin is arranged in a bundle and the organisation of myosin leaves no room for chance - motors can only contract. In some other cells where actin is disorganised, contraction would then come from this emergence phenomenon. “The primary function of a muscle cell is to contract in a given direction. The cost of creating an organised actin bundle is therefore worthwhile because of its efficiency. However, when some cells move, they assemble and disassemble their structures along the way. In these cases, a disorganised system with an actin network is a quick but crude solution suited to this fast-changing behaviour,” suggests Martin Lenz by way of explaining the different ways of producing a contraction which a living organism may implement.
The researcher is now developing experimental protocols to better understand the importance of buckling in emergent contraction. Their understanding opens up fields of research which go beyond that of cellular actin, such as wound healing processes where several cells contract in unison Besides biology, buckling is also involved in the extension of certain materials. The grain ‘buckles backwards’ and the effort is lost in the stretch when the grains separate. Although initially specific, the results obtained during the MicMactin project have been applied to much more diverse physical principles and have revealed some unexpected insights.

Publications:
- Lenz M. Reversal of contractility as a signature of self-organization in cytoskeletal bundles. eLife. 2020;9:e51751.
- Ronceray P, Broedersz CP, Lenz M. Fiber networks amplify active stress. PNAS. 2016;113(11):2827-2832.
- Lenz M, Thoresen T, Gardel ML, Dinner AR. Contractile Units in Disordered Actomyosin Bundles Arise from F-Actin Buckling. Phys Rev Lett. 2012 ;108(23):238107.