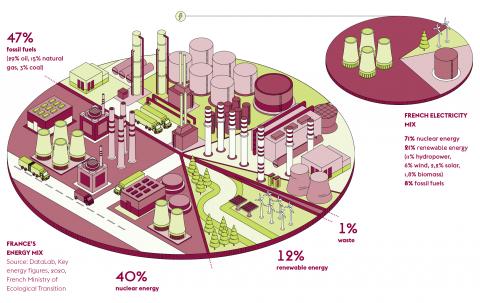
When the mix changes
(Article from l'Edition n.15 - february 2021)
Changes in France’s energy and electricity mix are being studied by researchers at Université Paris-Saclay, who are weighing up the flexibility of nuclear power, the fluctuation of renewable energies and the rise of hydrogen and nuclear cogeneration.
So many elements make up a country’s energy needs and guide its energy policy, such as how we move, stay warm and produce, and the means by which we do this. France’s energy mix is in the midst of transition in order to combat global warming and as a result of the French government’s commitments to energy conservation and carbon neutrality by 2050. With the law on the energy transition for green growth, which was passed on 17 August 2015, and the resulting multi-annual energy programme, France, its citizens, businesses, regions and public authorities are moving towards a new energy model.
The goals are ambitious: the country must reduce its consumption of fossil fuels (gas, coal, oil) – which emit greenhouse gases – by 40% by 2030 compared to 1990, and by 50% by 2050 compared to 2012 (16.5% by 2028). At the same time, the share of renewable energies in the production of electricity should rise to 40% by 2030 and that of nuclear energy should fall to 50% by 2035, with the closing of 12 of the 56 reactors currently operating in the country. These are daunting, challenging goals and are being studied by some of the researchers at Université Paris-Saclay, who are providing predictive models and methodologies to support these changes.
A carbon-neutral mix
With its many nuclear facilities, France currently has an energy mix which is already highly carbon neutral. Most of the energy it produces is nuclear – up to 77% of its primary energy production – and far outstrips hydropower energy and renewable and thermal energies, and exceeds that of coal. For its energy needs related to the production of electricity, transport fuels, heating or cooling of housing and industry, the country consumes 40% nuclear energy, 47% fossil fuels (oil 29%, natural gas 15%, coal 3%), 12% renewable energies (wood energy and bioenergy, hydropower, wind power, heat pumps) and waste (1%). Nuclear power is also one of the main components of the French electricity mix. 71% of the total electricity produced (547 TWh in 2019) comes from nuclear power plants, far in excess of the 21% provided by renewable energies (hydro 11%, wind 6%, solar 2.2%, biomass 1.8%) and the 8% of thermal energy from fossil fuels (gas, coal, fuel oil). With this kind of electricity mix, “the average greenhouse gas emissions for France are around 50 g of CO2 emitted per kilowatt-hour (kWh) of electricity produced,” points out Alain Le Duigou, a researcher at the Institute of Technicoeconomics of Energy Systems (I-Tésé – Univ. Paris-Saclay, CEA). Since some renewable energies are intrinsically intermittent, their increasing share in the final energy mix, as requested by the State, requires some thought on how to compensate for future variations in production (when it is dark, solar energy produces nothing and the same goes for wind energy when there is no wind) in order to continue to meet the country’s energy needs. “As we still have an ‘electricity whenever we want’ mindset, we need systems which respond to that demand,” says Alain Le Duigou. Could nuclear power plants play this role, even though they are said to be inflexible? Which drivers should be used?
The flexibility of France’s nuclear power plants
Commissioned between 1978 and 2002, the 56 reactors in France’s 19 nuclear power plants use pressurised water technology. The 32 oldest provide a maximum electrical power of 900 MW (the 12 reactors due to be closed are of this type). The four most recent ones provide 1,450 MW. The 20 other reactors provide a maximum of 1,300 MW and the future Flamanville EPR should attain 1,650 MW.
Here is a quick recap – the production of nuclear electricity is the result of a chain reaction which takes place in the heart of the reactor. This nuclear fission breaks apart heavy atomic nuclei, such as uranium 235 or plutonium 239, which are used as nuclear fuel. When a neutron collides with one of these nuclei, it splits into two lighter nuclei, releasing heat and a few neutrons, which then collide with other heavy nuclei and so on. In the reactor, a moderator, control rods and dissolved elements (boron in particular) modulate the speed at which neutrons disappear or are produced, and control the reaction. The heat released is transported out of the reactor by a heat transfer fluid and transferred to a closed primary circuit. A steam generator uses this heat to transform the liquid water in a secondary circuit, also closed, into steam. This steam goes to power a turbine and an alternator produces electricity. A condenser, fed by an independent cooling circuit, finally transforms the steam back into liquid water.
When they are not shut down for maintenance operations, nuclear power plants operate either at full power or under load monitoring, depending on how they are controlled. Using driving mode A, which does not allow large power variations to be achieved quickly, the oldest plants (900 MW) still operate at a given power level. The most recent ones, notably the 1300 MW reactors, equipped with driving mode G have load monitoring. Their power varies during the day according to a predefined programme, such as the 6/18 day/night power transient. It is RTE, France’s electricity transmission system operator, which sets the national power programme for nuclear power plants in France, and it is EDF, France’s leading electricity producer and supplier, which decides how to distribute this programme among its various reactors.
From optimisation to load monitoring
“Several other power transient profiles exist, but the main interest of the research is to see how to vary these scenarios, which are likely to be insufficient in the future,” says Valentin Drouet, whose thesis work was supervised by Jean-Michel Do from the “Service d’études des réacteurs et de mathématiques appliquées” (Department of Reactor Studies and Applied Mathematics) (SERMA – Univ. Paris-Saclay, CEA), with a focus on the optimisation of nuclear reactor management. While the future Flamanville EPR was designed to optimise its load tracking capacity, this is not the case for the rest of the nuclear power plants in France. In order to succeed in adapting existing power plants to the latest energy challenges, two options are possible.
The first is to act directly at a plant level and identify the parameters on which to focus in order to improve the control mode’s performance indicators. Load monitoring, which is part of a plant’s normal operating margins, also has a cost, i.e. direct, maintenance and safety costs, and any variation generates additional costs. Varying the power of a power plant more often implies, for example, a larger volume of radioactive effluents, which will then have to be treated. “More intense load monitoring can also increase the ageing of certain materials, particularly the control rods, which move more frequently back and forth to the core of the plant to adjust the power,” adds the young doctor. Thermal fatigue phenomena cannot be excluded either. During large variations in power and temperature, materials are subject to mechanical stress due to differential expansion – an expansion delay between the fuel and the casing. “The reactor can also accumulate xenon, a neutron poison which reduces the chain reaction and whose presence is normally balanced by diluting the boron. However, a reactor at the end of a campaign has a lower boron concentration and its room for manoeuvre is more limited,” concludes Jean-Michel Do.
A more effective algorithm
Finding the best compromise between cost and power stability is therefore no easy task for researchers. To achieve this, Valentin Drouet and his colleagues are using multiphysics simulation. “A multi-objective optimisation algorithm has been developed, based on a reactor simulator, which evaluates reactor performance for each new configuration of control rod management parameters.” From the family of evolutionary algorithms, it reproduces the natural evolution of species. “It is a stochastic algorithm, which makes it possible to effectively explore the margin of optimisation of the control rod management.” Calculations show that a power plant’s best performance is obtained by increasing the width of the operating range of the temperature control unit, among other things. “By increasing the stability of the reactor, we also increase its availability. It becomes capable of chaining several power transients together more quickly.” The result is increased flexibility.
The SERMA team’s field of research has now widened as it is tackling the optimisation of the power programme distribution across all nuclear power plants in France, which is the second possible option for improving the flexibility of nuclear power. “Depending on the reactors and their stage in their cycle, they are not all able to withstand the same power variability. We are going to need even better optimisation techniques,” points out Jean-Michel Do.
Clarifying goals using prospective scenarios
The fluctuating nature of renewable energies and nuclear flexibility are issues which are the focus of studies and models being carried out by the researchers of the team “Économie durable” (Sustainable economy) from the “Laboratoire génie industriel” (Industrial Engineering Laboratory) (LGI – Univ. Paris- Saclay, CentraleSupélec) in collaboration with I-Tésé. These scenarios take into account physical data about wind and sunlight, the evolution of the efficiency of the different technologies, the extension of the lifespan of nuclear power plants or their replacements and the costs generated, etc.
By contrasting theory and practice, the team can confirm the potential for the flexibility of nuclear power at the level of the reactor and also at the level of all nuclear power plants in France, with 40% of current reactors already carrying out load monitoring. Even better, they can show the room for manoeuvre. “It has been estimated that 30% of the need for flexibility in response to the variability of renewables could be met by nuclear power by 2050,” says Pascal Da Costa, who is in charge of the Sustainable economy team at LGI. “This is because if, at the level of a power plant, the reactor’s behaviour shows a certain amount of inertia, on the scale of all the nuclear power plants in France it becomes possible to achieve significant power variations in a short period of time by coordinating the different reactors.” But, where can the remaining 70% be found? “Probably in areas other than energy production, such as increasing the energy efficiency of buildings. With better insulation, heating requirements are reduced,” suggests Pascal Da Costa. “However, if the nuclear power plants in France do not want to benefit from flexibility, then, in case of overproduction of electricity by renewable energies, it will be necessary to find another outlet for this excess of energy,” continues Pascal Da Costa. This could be an opportunity to produce hydrogen for example, which still does not feature largely in the French energy mix, and only if its economic benefit is proven. In long-term projections, hydrogen often appears as a source of flexibility and a means of reducing greenhouse gas emissions. But what does this actually mean?
What are the newcomers in the mix?
Currently, France produces 450,000 tonnes of hydrogen per year by steam methane reforming, which results in an emission of around five megatonnes of CO2. At the same time, coproduction by other processes results in 500,000 tonnes of hydrogen. All of it is used in oil refining (60%), ammonia and ammonia fertiliser production (25%), the chemical industry (10%), metallurgy (1%) and other more diverse uses (4%). However, a production method based on the electrolysis of water is possible. As long as the electrolysers are supplied with very low-carbon electricity, such as from nuclear and renewable energies, it would help to reduce CO2. “The market for such a scenario has been evaluated. The economic cost of producing hydrogen using nuclear electricity depends on who owns the electrolysers. It is less if it is EDF rather than another key player, who would first have to buy back the electricity to run the electrolysers,” points out Pascal Da Costa.
Among the multitude of possible applications of a very low-carbon hydrogen, the most promising markets are electric mobility, as a synthetic fuel, and gas to be burned. “If we switched the entire fleet of land vehicles to hydrogen, we would need 8 million tonnes of hydrogen per year in France. This would avoid importing the equivalent of 40 million tonnes of oil,” says Alain Le Duigou. Internal combustion engines, kept on a temporary basis, could support a transitional period of massive hydrogen production aimed at the manufacture of synthetic fuels. However, replacing the carbon-based technology currently on the market with new low-carbon hydrogen systems, which still remain expensive today, would require significant investment. “Both industrial and political action will be needed to develop production and distribution facilities through, for example, incentive and taxation schemes to reduce the cost of hydrogen at the pump,” says Alain Le Duigou.
If, by 2035, hydrogen is not indispensable as a means of storage to diversify the electricity mix and support an increase in renewable energy, this will change by 2050, when scenarios are based almost exclusively on these energies. Hydrogen would then contribute to the balance of the electricity grid by providing a storage and destocking solution, whether for transport or stationary use. “The combination of a lithium battery and a fuel cell would be an interesting option,” points out Alain Le Duigou.
As with hydrogen, the question of cogeneration – the simultaneous production of two energies – in the energy mix is a matter of debate. Today, it is estimated that only one third of the energy from pressurised water reactors is converted into electricity, with the rest ending up in the environment. Nuclear cogeneration would recover this lost energy in the form of heat and reinject it into the nearby district heating network via new “piping”. “The pipes which carry the heat have recently become more technologically advanced and are now more energy efficient. This favours cogeneration,” points out Pascal Da Costa.
With the development of the mix, traditional energy markets are changing, new roles are appearing and are making their mark. See you in 2050 for the final outcome.
Publications
- Datalab: Key figures for energy, 2020 Edition from the Ministry of Ecological Transition.
- V. Drouet, S. Verel, and J.-M. Do. Surrogateassisted asynchronous multiobjective algorithm for nuclear power plant operations. In Proceedings of the 2020 Genetic and Evolutionary Computation Conference GECCO’20, (2020).
- C. Cany, et al. Nuclear power supply: Going against the misconceptions. Evidence of nuclear flexibility from the French experience, Energy, 151, (2018).
- Olfa Tlili, et al. Geospatial modelling of the hydrogen infrastructure in France in order to identify the most suited supply chains, International Journal of Hydrogen Energy, 45 (4), (2020).